Cows produce powerful HIV antibodies
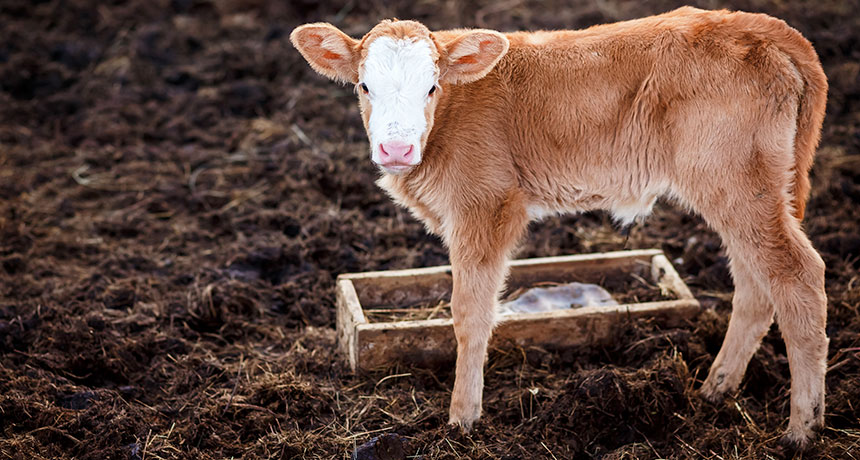
An unlikely hero has emerged in the quest to fight HIV: the cow. In a first for any animal, including humans, four cows injected with a type of HIV protein rapidly produced powerful antibodies against the virus, researchers report. Learning how to induce similar antibodies in humans may be key to a successful HIV vaccine.
The antibodies, called broadly neutralizing antibodies, can stop infection from a variety of HIV types. The cows generated these antibodies as soon as 42 days after immunization, the researchers report online July 20 in Nature. For the small percentage of people estimated to develop these antibodies after a natural infection, it can take several years.
The work identifies “a new and much more efficient method to generate broadly active antibodies against HIV,” says immunologist Justin Bailey of Johns Hopkins University School of Medicine, who was not involved in the study.
Making an HIV vaccine has proved difficult because the virus changes all the time. Different strains exist throughout the world, and the virus even mutates within an infected person’s body. Most often, people develop antibodies that are specific to one strain but ineffective against others. HIV vaccines tested so far have not led to the production of broadly neutralizing antibodies.
About 1 percent of HIV-infected people eventually generate broadly neutralizing antibodies that are especially potent and effective against many types of HIV. The development of these antibodies doesn’t seem to help infected people. But when given to monkeys before exposure to a virus similar to HIV, the antibodies prevent infection.
Broadly neutralizing antibodies specific to HIV have a few quirky features, one of which is the presence of a long stretch of amino acids that sticks out from the antibody surface. This protruding part of the antibody binds to a viral site that remains the same between strains, because the virus needs it to gain entry to a cell. HIV’s thick coat of surface sugars makes the viral binding site difficult to access. A longer stretch of amino acids seems to be able to pierce through “and reach in, almost like the long arm of the law,” says Vaughn Smider, a molecular immunologist at the Scripps Research Institute in La Jolla, Calif.
In people infected with HIV who develop broadly neutralizing antibodies, this antibody region — called HCDR3 — has about 30 amino acids, about twice as long as what is usual for human antibodies. Although on the long side for a human, “that’s actually kind of short for a cow,” Smider says.
And so the idea to immunize cows was born. Since cows naturally make longer HCDR3s, Smider explains, perhaps they’d have this sought-after response to HIV.
Smider and colleagues took serum — blood with the cells removed, leaving antibodies behind — from four immunized cows and tested it against different types of HIV virus in a test tube. All of the cows developed broadly neutralizing antibodies. The researchers then tested one cow’s antibodies on an even larger number of virus types. After 381 days, this cow’s antibodies prevented 96 percent of the 117 HIV types from infecting cells in a lab dish. The researchers also isolated an antibody from this cow that had a long HCDR3 of 60 amino acids and stopped infection by 72 percent of the HIV types.
If researchers could induce antibodies with long HCDR3s in humans, “then that could be the basis of getting a vaccine to work,” Smider says. “You need a step before the immunization that helps expand the rare antibodies.” Since cows are so good at making broadly neutralizing antibodies, it also might be possible to turn the cow’s handiwork into drugs for HIV treatment, if bovine antibodies are effective at stopping the virus in other animals, he says.