Toddlers’ screen time linked to speech delays and lost sleep, but questions remain
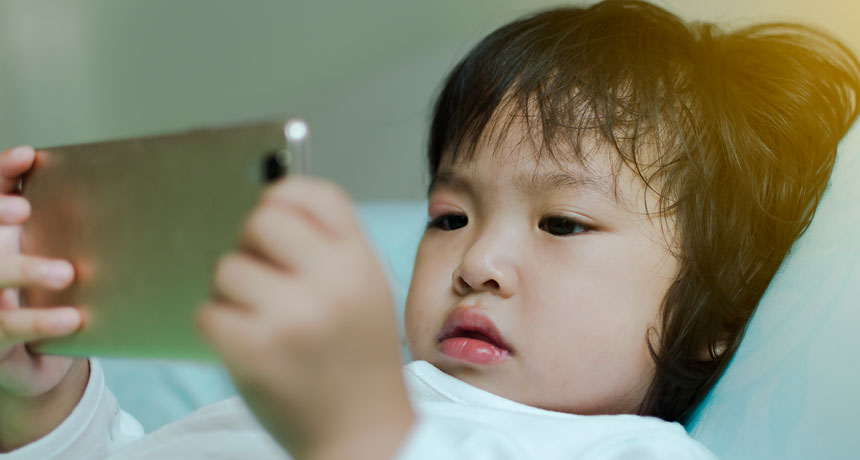
One of the most pressing and perplexing questions parents have to answer is what to do about screen time for little ones. Even scientists and doctors are stumped. That’s because no one knows how digital media such as smartphones, iPads and other screens affect children.
The American Academy of Pediatrics recently put out guidelines, but that advice was based on a frustratingly slim body of scientific evidence, as I’ve covered. Scientists are just scratching the surface of how screen time might influence growing bodies and minds. Two recent studies point out how hard these answers are to get. But the studies also hint that the answers might be important.
In the first study, Julia Ma at the University of Toronto and colleagues found that, in children younger than 2, the more time spent with a handheld screen, such as a smartphone or tablet, the more likely the child was to show signs of a speech delay. Ma presented the work May 6 at the 2017 Pediatric Academic Societies Meeting in San Francisco.
The team used information gleaned from nearly 900 children’s 18-month checkups. Parents answered a questionnaire about their child’s mobile media use and then filled out a checklist designed to identify heightened risk of speech problems. This checklist is a screening tool that picks up potential signs of trouble; it doesn’t offer a diagnosis of a language delay, points out study coauthor Catherine Birken, a pediatrician at The Hospital for Sick Children in Toronto.
Going into the study, the researchers didn’t have expectations about how many of these toddlers were using handheld screens. “We had very little clues, because there is almost no literature on the topic,” Birken says. “There’s just really not a lot there.”
It turns out that about 1 in 5 of the toddlers used handheld screens, and those kids had an average daily usage of about a half hour. Handheld screen time was associated with potential delays in expressive language, the team found. For every half hour of mobile media use, a child’s risk of language delay increased by about 50 percent.
“The relationship is not that strong,” Birken says, and those numbers come with big variations. Still, a link exists. And finding that association means there’s a lot more work to do, Birken says. In this study, researchers looked only at time spent with handheld screens. Future studies could investigate whether parents watching along with a child, the type of content or even time of day might change the calculation.
A different study, published April 13 in Scientific Reports, looked at handheld digital device use among young children and its relationship to sleep. As a group, kids from ages 6 months to 3 years who spent more time using mobile touch screen devices got less sleep at night.
Parent surveys filled out online indicated that each hour of touch screen use was linked to 26.4 fewer minutes of night sleep and 10.8 minutes more sleep during the day. Extra napping time “may go some way to offset the disturbed nighttime sleep, but the total sleep time of high users is still less than low users,” says study coauthor Tim Smith, a cognitive psychologist at Birkbeck, University of London. Each additional hour of touch screen use is linked to about 15 minutes less sleep over 24 hours.
By analyzing 20 independent studies, an earlier study found a similar link between portable screen use and less sleep among older children. The new results offer “a consistent message that the findings from older children translate into those younger,” says Ben Carter of King’s College London, who was a coauthor on the study of older children.
So the numbers are in. Daily doses of Daniel Tiger’s Neighborhood on a mobile device equals 7.5 minutes less sleep and a 50 percent greater risk of expressive language delay for your toddler, right? Well, no. It’s tempting to grab onto these numbers, but the science is too preliminary. In both cases, the results show that the two things go together, not that one caused the other.
It may be a long time before scientists have answers about how digital technology affects children. In the meantime, you can follow the American Academy of Pediatrics’ recently updated guidelines, which discourage screens (except for video chatting) before 18 months of age and for all children during meals or in bedrooms.
We now live in a world where smartphones are ever-present companions, a saturation that normalizes the sight of small screens in tiny hands. But I think we should give that new norm some extra scrutiny. The role of mobile devices in our kids’ lives — and our own — is something worth thinking about, hard.