It’s best if babies don’t drink their fruit as juice
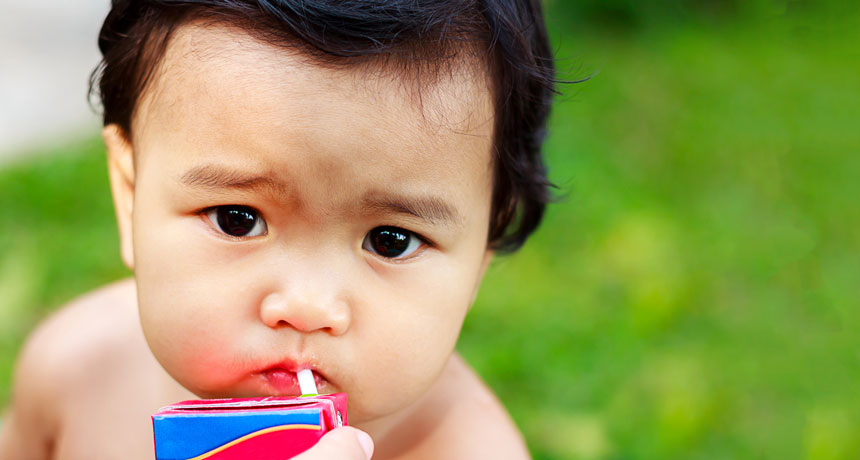
The juice saga continues. The American Academy of Pediatrics updated their official ruling on fruit juice, recommending none of the sweet stuff before age 1. Published in the June Pediatrics, the recommendation is more restrictive than the previous one, which advised no juice before age 6 months.
The move comes from the recognition that whole fruits — not just the sweet, fiberless liquid contained within — are the most nutritious form of the food. Babies under 1 year old should be getting breast milk or formula until they’re ready to try solid foods. After their first birthdays, any extra liquids they drink should be water or milk. (These updated guidelines may not apply to babies who might need fruit juice to help with constipation.)
Whole fruits — or, mashed up clumps of them — have more fiber and protein than juice. The only benefit that juice has over its former whole form is that it’s way easier for a kid to slurp down.
The potential risks of cavities and obesity in part prompted the updated guidelines. It’s worth saying that neither of these outcomes are guaranteed with juice drinking. In fact, a recent study failed to find a link between juice drinking and excessive weight gain in children. Still, juice offers no nutritional advantage over whole fruits, so the reasoning of the AAP seems to be, “Why risk it?”
The AAP gave additional, more nuanced advice for parents who do decide to give juice to children age 1 and older:
Don’t give kids juice in bottles or sippy cups, especially at bedtime. That ease of drinkability would encourage kids to drink juice for long periods of time, prolonging sugar baths for teeth.
Look out for unpasteurized juice. Harmful forms of E. coli bacteria can appear in unpasteurized apple cider, for instance, posing a particular risk for young people.
Give 1- to 3-year-old kids no more than 4 ounces of juice a day. That recommendation drops 2 ounces from earlier guidance that limited juice to between 4 and 6 ounces daily. Children ages 4 to 6 years old get those extra 2 ounces back, with a daily limit of between 4 and 6 ounces.
Make sure kids are drinking 100 percent juice, not those sneaky “cocktails” or “drinks.” Those are often nutritional wastelands, packed with even more sugar and devoid of other nutrients.
Though the guidelines don’t mention habit formation, I suspect this also came into play in the AAP’s encouragement of whole fruits over juice. Children’s taste preferences get shaped early. Really early, actually. Fetuses learn to love flavors their mothers ate while pregnant. So babies who grow accustomed to sweet juice might be less impressed with water or milk. And while that might not be a problem in the early years of life, years or decades of drinking sweet liquids will catch up with them eventually.