For glass frogs, moms matter after all
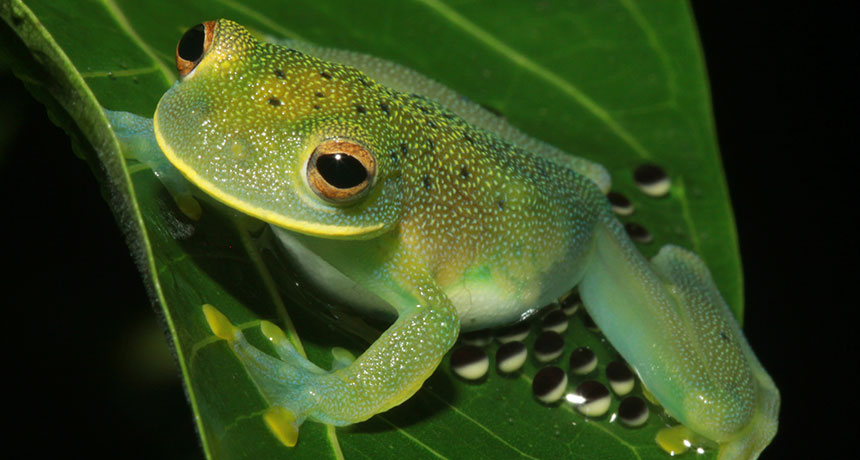
Glass frogs often start life with some tender care from a source scientists didn’t expect: frog moms.
Maternal care wouldn’t be news among mammals or birds, but amphibian parenting intrigues biologists because dads are about as likely as moms to evolve as the caregiver sex. And among New World glass frogs (Centrolenidae), what little parental care there is almost always is dad’s job — or so scientists thought, says Jesse Delia of Boston University.
Months of strenuous nights searching streamside leaves in five countries, however, have revealed a widespread world of brief, but important, female care in glass frogs. In examining 40 species, Delia and Laura Bravo-Valencia, now at Corantioquia, a government environmental agency in Santa Fe de Antioquia, Colombia, found that often mothers lingered over newly laid eggs for several hours. By pressing maternal bellies against the brood, moms hydrated the jelly-glop of eggs and improved offspring chances of survival, Delia, Bravo-Valencia and Karen Warkentin, also of BU, report online March 31 in the Journal of Evolutionary Biology.
Glass frogs take their name from see-through skin on their bellies and, in certain cases, transparent organ tissues. (Some have clear hearts that reveal blood swishing through.) These frogs aren’t exactly obscure species, but until this field project, which stretched over six rainy seasons, female care in the family was unknown.
Female glass frogs may not cuddle their eggs for long, but it’s enough to matter, the researchers found. As is common in frogs, the mothers don’t drink with their mouths but absorb water directly through belly skin into a bladder. Moms pressing against a mass of newly laid eggs caused the protective goo to swell — perhaps by osmosis or peeing — and the mass to quadruple in size. For some of the glass frogs in the study, the youngsters were on their own once mom left. But at least hydration created an unpleasant amount of slime for a predator to bite through before getting to frog embryos.
Night-hunting katydids in captivity, when offered a choice, barely nibbled at a hydrated mass of frog offspring, concentrating instead on eating an unhydrated clutch. In the field, when researchers removed about two dozen moms from their clutches in two species, mortality at least doubled to around 80 percent. Predators and dehydration caused the most deaths.
There are still more than 100 glass frog species that Delia and Bravo-Valencia haven’t yet watched in the wild. But the researchers did track down maternal care in 10 of 12 genera. Such a widespread form of maternal care probably evolved in the ancestor of all glass frogs, the researchers propose after analyzing glass frog family trees several ways.
In contrast, prolonged care from glass frog dads — rehydrating the egg mass as needed and fighting off predators such as hungry spiders — seems to have arisen independently later, at least twice. Across evolution in the animal kingdom, “usually we don’t see transitions from female to male care,” Delia says. “The pattern we found is completely bizarre.”
Why females started hanging around their eggs at all fascinates Hope Klug, an evolutionary biologist at the University of Tennessee at Chattanooga who studies parental care. In frogs, with eggs mostly fertilized externally, females could easily leave any care to dad.
“Parental care is perhaps more common and diverse in animals than we realize,” she says. “We just might have to look a little bit harder for it.”